A section from higher in the core at (9977 ft) 3041 m also contains
several large and highly fractured plagioclase phenocrysts (Figs.
S-8 and S-9). The basal 8 m of the core
contains many small rock and mineral clasts in a predominately glassy
matrix that has partially altered to chlorite. Brownish glass shows evidence
of flow structure (Fig. S-10) and calcite
is observed as veins and in cavities in several thin sections (Fig. S-11,
S-13, S-14).
The mineral clasts are mostly single and multiple aggregates of plagioclase
and the lithic clasts are of glassy fragments. The complex mixtures and
very different textures in the lower 8 m of the core are similar to the
cores from inside the Chicxulub crater (23-26, Figs. 4,
S-3, S-9,
S-10, S-11,
S-12, S-13,
S-14).
The clast from 3044 m (9986 ft.) contains shocked minerals surrounded
by a matrix that is almost entirely glass except where it has been altered
to chlorite. The samples include shock-melted plagioclase that has been
completely or partially converted to glass (Figs. 6
and 8), spherulitic glass (Figs. 7a
and 7b) and pure silica glass (SiO2)
(Fig. 7c). Plagioclase encloses diaplectic
glass (maskelynite) with a composition identical to the surrounding plagioclase
(An50) (Fig. 8, analyses 3 and 4 in
Table S-1). We also identified magnesian iron titanium oxide [(Fe.84,
Mg.14) TiO3] grains that are stoichiometrically ilmenite, heterogeneous
silica glass, albite, sanidine and a partially melted carbonate (CaCO3)
clast with fragmented ooids (27-28) (Figs. 5,
6, 7,
8, S-4 to S-7ab and
Table S-1). Sanidine,
identified optically and confirmed by microprobe analysis of a 10-mm grain
(analysis 5 in Table
S-1) has 43% albite in solid solution without any sign of segregation
(microperthite).
The Lagrange-1 cuttings consist of various types of partly crystalline
and partly glassy rock of mostly basaltic composition (Fig. S-7). Some
of the fragments are identical to those found in the Bedout core (see
Figs. S11-S15). One of these fragments, sample LG-3255 m (10,679 ft.),
is shown in Fig. (S-7a,b). Fig. S-7a shows feldspar crystallites (laths)
in “swallowtail” terminations, indicating rapid crystallization
from the glassy matrix. The feldspar laths display heterogeneous compositions,
and are mixtures of either pure albite (Table
S-1 #1) or K-feldspar (Table
S-1 #2) in their glassy matrix (Table
S-1 #3) as seen in the back-scattered image (S-7b) of one of the grains.
We interpret these textures, chemistry, mineralogy, and mixture of different
fragments as indicating that the basal 8 m of Bedout-1 is an impact melt
breccia. The completely or partially melted and fractured plagioclase
crystals and abundant glassy clasts are most diagnostic. The coexistence
of titanium-rich silica glass in close proximity (within 1 mm) to titanium-poor,
but slightly aluminous silica glass (analysis 23 and 24 in Table
S-1, Fig. 7c) requires silicate
liquid immiscibility that is not seen in terrestrial magmatic environments.
Partially melted and re-crystallized carbonate lithic fragments (Fig.
5) and spherulitic glasses (partially altered to chlorite, Fig.
7b and analysis 22 in Table
S-1), with a different chemical composition from the glassy matrix,
are again features attributable to an impact-generated melt breccia. Magnesian-ilmenite
(analysis 20 in Table
S-1) found as microlites in the matrix is also an uncommon mineral
in volcanic rocks. The glassy rock clasts can be attributed to melting
of target materials that contain Mg-rich sediments (e.g. dolomites) and
common Fe-Ti oxides (e.g. magnetite, titanite ‘sphene’ and
rutile), found in crustal environments. Overall, the composition of the
minerals and glasses of the Bedout core are consistent with a heterolithic
impact breccia or melt-rich suevite, formed by impact triggered heterogeneous
melt formation and subsequent quenching and crystallization. Such compositions
are unknown and unlikely to exist in terrestrial volcanic agglomerates,
lava flows and intrusive pipes. Individually, some of these minerals may
rarely occur in volcanic or plutonic rocks, but never in association with
each other.
In particular, natural volcanic processes generate silicate melts up
to but not exceeding about 78% silica. Taken as a whole, these features
are most consistent with impact-generated melting. Volcanism associated
with rifting (nor any other endogenous magmatic process) does not produce
melts (glasses) like (#21) and (#22) in Table
S-1. The overall textures of these heterolithic fragments, especially
the Bedout glasses, are similar to the features of the Sudbury Onaping
breccia and the melt breccias inside the Chicxulub crater (Fig.
4) (22-25).
Ar/Ar Dating of the Bedout Core
We undertook 40Ar/39Ar age measurements on feldspar concentrates from
the Bedout-1 core and Lagrange-1 impact breccia by ‘step-heating’
and single crystal fusion experiments (29, also see SOM). 40Ar/39Ar dates
on six individual plagioclases 3041 m (9977 ft.) from the Bedout-1 core
indicate ages that are much younger than the overlying Triassic sediments.
Petrographic and microprobe examination of the Bedout core 3044 m and
3041m (9986 ft. and 9977 ft.) revealed significant alteration in plagioclase
grains and possibly extensive K addition (Figs.
6-8 and S-8, S-9) resulting in young
40Ar/39Ar ages. Individual feldspar grains display heterogeneous chemical
compositions due to alteration or disequilibrium in the sample cuttings
(Table S-1, Figs
S-7a, S-7b).
The glassy matrix from 3044 m (9986 ft.) had extremely low K (<0.1%)
and proved unsuitable for 40Ar/39Ar dating. A plagioclase separate at
3255 m (10,679 ft.) from the Lagrange-1 cuttings, that displayed the least
evidence of alteration or disequilibrium, has an 40Ar/39Ar age of 250.2
with a plateau portion between 8% and 90% gas release at 250.1 ±
4.5 Ma (1s), (S-17, S-18, S-19)
consistent with the previous K-Ar measurement on a plagioclase separate
from Lagrange-1 (253 ± 5 myrs; 30) (Fig. 9,
SOM). Similar problems in dating plagioclase separates were observed in
the Yucatan-6 melt rocks from the Chicxulub crater (24).
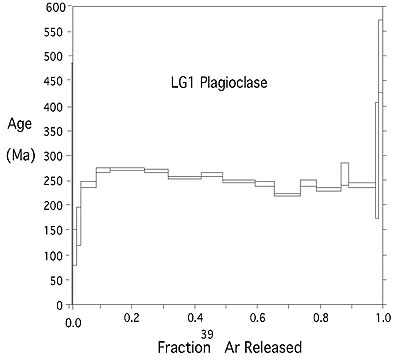 |
Figure 9. Ar/Ar step-heating
ages for the Lagrange-1 plagioclases from the top of the Bedout High
3255 m (10,679 ft.) indicate an age of 250.1 +/- 4.5 myrs. Click
here for a larger view |
|